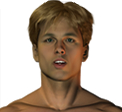
James Bailey
21, Male
Questions: Explain the following examination findings
Click on each title below to see the model answer...
Q1. James is tachypnoeic (high respiratory rate). What are the potential causes?
Model answer:
Tachypnoea indicates that the drive to breathe (neural respiratory drive) has increased.
Potential causes of increased neural respiratory drive in acute asthma include:
- Hypoxia (SpO2 91%, peripherally and centrally cyanosed); peripheral chemoreceptor activation.
- Increased work of breathing (lung, airway chest wall & skeletal muscle mechanoreceptors):
- bronchoconstriction in asthma increases airway resistance (RAW).
- There is also lung hyperinflation and gas trapping if bronchoconstriction is severe; this also impairs respiratory mechanics & increases work of breathing.
Residual volume (RV) increases due to premature airway closure during full expiration, as a result of increased smooth muscle tone, oedema & inflammation of the airway walls, and abnormal secretions. As a result airflow stops and air gets trapped in these collapsed airways – there is “gas trapping” or “lung hyperinflation”. Airway pressure may become positive at end-expiration as a result (positive end-expiratory pressure – “PEEP”).
As illustrated in the diagram below, an increase in RV increases end-expiratory lung volume (EELV) and pushes functional residual capacity higher and closer to TLC (total lung capacity). Because FRC is now closer to FRC, there is now a reduced inspiratory reserve volume (IRV), which limits tidal volume expansion (there is less “room” to breathe in). The only other way of increasing minute ventilation is by increasing respiratory rate (VE = VT x Vf).
Figure 1: Lung hyperinflation reduces inspiratory reserve volume (IRV) & limits tidal volume expansion The asthmatic is now also breathing at the less compliant portion of the lung pressure–volume curve so breathing in is harder work for the respiratory muscles. It becomes energetically more favourable to increase minute ventilation by increasing respiratory rate rather than trying to take a deeper breath in.
Q2. Review the main mechanisms of arterial hypoxaemia. What is the dominant mechanism of hypoxaemia in acute asthma?
Model answer:
Causes of arterial hypoxaemia: Hypobaric hypoxia, diffusion impairment, V/Q mismatch, right-to-left shunt, alveolar hypoventilation.
Ventilation-perfusion mismatch is the key mechanism of hypoxaemia in acute asthma: reduced ventilation relative to perfusion in lung regions where there is bronchoconstriction. Remember that in hypoxaemia due to V’/Q’ mismatch, the PaCO2 will be low - more CO2 will be being "blown off" from well-ventilated alveoli in the face of increased (hypoxic) respiratory drive.
Hypoventilation occurs in life-threatening cases: if there is alveolar hypoventilation, PaCO2increases. In an acute asthma attack, a low PaCO2 indicates that the patient is able to mount an appropriately high level of respiratory drive. Beware a normal or high PaCO2 in acute asthma: anything other than a low PaCO2 indicates that the ventilatory response is insufficient, and is a sign of a life-threatening asthma attack.
(Surprisingly there is no evidence of pure R to L shunt, even though asthmatics who come to autopsy have extensive mucous plugging. Explanation presumed to be collateral ventilation that reaches lung situated behind completely closed bronchioles).
Q3. What is the difference between the terms hypoxia and hypoxaemia?
Model answer:
Hypoxia refers to insufficient O2 at the cellular level. There are four general categories of hypoxia:
- Hypoxic hypoxia - characterised by a low arterial blood PO2, accompanied by inadequate Hb saturation.
- Anaemic hypoxia - refers to a reduced O2-carrying capacity of the blood.
- Circulatory hypoxia - arises when too little oxygenated blood is delivered to the tissues.
- Histotoxic hypoxia - characterised by normal O2 delivery to the tissue, but the cells are unable to use the oxygen available to them (cyanide poisoning).
Hypoxaemia refers to reduced oxygen tension in the blood (arterial hypoxaemia – low PaO2) (-aemia indicates “of the blood”).
Q4. Why is James peripherally and centrally cyanosed?
Model answer:
Cyanosis :
- If supply of O2to the tissues is deficient, content of de-oxyHb in tissue capillaries increases because of hypoxia.
- de-oxyHb has a blueish tinge, causing discolouration of the tissues = cyanosis.
- generally 2 types of cyanosis can occur – peripheral and central.
Peripheral cyanosis = bluish grey tinge to appear in extremities (e.g. hand, feet). This can be caused either by reduced blood flow resulting in reduced oxygen delivery, and therefore hypoxic, to under-perfused tissue, or by hypoxaemia.
Causes of reduced blood flow:
- cardiovascular shock
- low temperature
- reduced cardiac output
- poor arterial supply
Under the above conditions respiration is OK, and arterial O2 content probably also normal.
In central cyanosis:-
There is arterial hypoxaemia (reduction in O2 content) –buccal mucosa and lips are the best sites to spot this.
If arterial blood contains > 1.5 -2 g.dl-1of deoxyHb, cyanosis is observable even in well-perfused tissues. Occurs when HbO2 saturation < 85% if [Hb] normal (15 g.dl-1).
Q5. The absence of focal signs in the chest helps to exclude pneumonia and pneumothorax. Does the absence of wheeze exclude asthma?
Model answer:
No. Wheezing sounds are generated by oscillations of opposite walls of airways when they are obstructed and near the point of closure. If there is not enough airflow because of the severity of the narrowing wheezing sounds will not be produced. Thus while in moderate asthma there is usually wheezing, in severe attacks, the chest may be quiet, with scarcely audible breath sounds. Hence the ominous silent chest in very severe asthma. There will often also be a previous history of episodes of wheezing.
Q6. What is the definition of pulsus paradoxus? Why does it occur during a severe asthma attack?
Model answer:
There is a normal physiological fall in systolic blood pressure up to 10 mmHg during inspiration. Pulsus paradoxus is the exaggeration of this normal decline in systolic blood pressure more than 10 mmHg during inspiration. The “paradox” refers to the fact that heart sounds may be heard over the precordium when the radial pulse is not felt.
Mechanisms in asthma:
-
The first is a direct consequence of the reduction in pleural pressure associated with inspiration (which becomes exaggerated during an asthma attack).
Since all the organs in the thorax are exposed to changes in pleural pressure, their function is unaffected, except where arteries leave or veins enter the thoracic cage. Here, a change of intrathoracic pressure alters the pressure gradients along which blood leaves or enters the thorax.
Since the walls of the heart are distensible, a reduction of intrathoracic pressure, such as during inspiration, will be the equivalent of lowering the pressure within the heart and lungs relative to the systemic arterial and venous pressures outside the thorax. There is then an increase in the force that the left ventricle must develop in the next beat to sustain the same arterial pressure; that is, the left ventricle experiences increased afterload and empties less completely and systemic pressure falls until compensatory mechanisms can restore it.
This mechanism contributes to pulsus paradoxus both in normal breathing and in pathologic states. In normal breathing, its effect is slight, but in the forced breathing of an asthmatic attack, it may be the principal cause of the substantial respiratory fluctuation of arterial pressure that is sometimes observed.
The second mechanism is a consequence of the intimate anatomic relation between the two ventricular chambers such that distension of one influences the filling characteristics (distensibility, or apparent compliance) of the other.
Reduction of pleural pressure also increases the gradient from the systemic venous reservoir to the right ventricle, and venous return is accelerated. The increase in the preload of the right ventricle that accompanies a fall in pleural pressure would not immediately affect left-ventricular function if the two ventricular chambers did not share a common wall and reside inside a single pericardial sac. A selective increase in right-ventricular-filling pressure lowers the trans-septal diastolic pressure gradient and shifts the septum leftward, thus immediately affecting the volume of blood that will be accepted by the left ventricle for any given pressure, that is, its apparent diastolic compliance. The resultant reduction of enddiastolic volume diminishes the stroke work of the next left-ventricular systole.